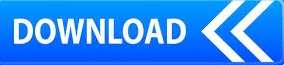
Conversely, inhibition of translation elongation and trapping of the mRNAs in polysomes lead to the loss of P-bodies ( S heth and P arker 2003 T eixeira et al. For example, blocking translation initiation using mutations in initiation factors leads to an increase in the P-body size and number along with accelerated decay rates. Analyses of P-bodies provide additional evidence for an inverse relationship between translation and formation of mRNPs capable of mRNA decapping. P-bodies are cytoplasmic foci that accumulate translationally repressed mRNA along with the decay machinery and translational repressors (reviewed in E ulalio et al. When mRNAs exit translation, they form nontranslating mRNPs, which can undergo decapping and degradation and/or accumulate in cytoplasmic foci referred to as P-bodies ( S heth and P arker 2003). The balance between translation and decay also correlates with the type of mRNP formed and its subcellular localization. Thus, a key step in mRNA decapping is exchanging translation initiation factors for the mRNA decapping machinery. Consistent with this competition, eIF-4E has been shown to inhibit the decapping enzyme in vitro ( S chwartz and P arker 1999). Moreover, mutation of initiation factors such as eIF-4E, the cap binding protein, or Prt1 (part of the eIF3 complex) lead to faster degradation of mRNAs ( S chwartz and P arker 2000). Conversely, mRNAs poorly translated because of cis elements, such as secondary structures in the 5′ untranslated region or a poor AUG context, are decapped faster than their well-translated counterparts ( M uhlrad et al. For example, when mRNAs are maintained in association with ribosomes by the inhibition of translation elongation using cycloheximide, the rate of decapping is reduced ( B eelman and P arker 1994). Moreover, many observations indicate that decapping and translation in yeast cells are intertwined processes that are often in competition. 1996 D unckley and P arker 1999).ĭecapping is a critical step in this decay pathway as it permits destruction of the mRNA and is a site of numerous control inputs. The 5′ m 7G cap is then removed by the Dcp1/2 decapping enzyme and 5′–3′ decay is performed by the exonuclease Xrn1 ( H su and S tevens 1993 B eelman et al. In Saccharomyces cerevisiae, the major pathway of mRNA decay involves decapping followed by 5′–3′ decay ( C oller and P arker 2004), with removal of the poly(A) tail predominantly promoted by the Ccr4/Pop2/Not deadenylase complex ( D ecker and P arker 1993 M uhlrad et al. In eukaryotic cells there are two general mechanisms for the degradation of mRNAs, both of which initiate with deadenylation, leading either to 3′–5′ exonucleolytic degradation or to decapping followed by 5′–3′ exonucleolytic destruction of the mRNA (reviewed in P arker and S ong 2004 G arneau et al. These results identify Stm1 as an additional component of the mRNA degradation machinery and suggest a possible connection of mRNA decapping to ribosome function.ĬONTROL of mRNA translation and degradation are important points of regulation of eukaryotic gene expression. Consistent with Stm1 affecting Dhh1 function, stm1Δ strains are defective in the degradation of the EDC1 and COX17 mRNAs, whose decay is strongly affected by the loss of Dhh1. Stm1 loss-of-function alleles and overexpression strains show several genetic interactions with Pat1 and Dhh1 alleles in a manner consistent with Stm1 working upstream of Dhh1 to promote Dhh1 function. To understand the function of these factors, we identified the ribosome binding protein Stm1 as a multicopy suppressor of the temperature sensitivity of the pat1Δ strain. In the budding yeast Saccharomyces cerevisae, decapping is promoted by the Dhh1 and Pat1 proteins, which appear to both inhibit translation initiation and promote decapping. mRNA degradation is often initiated by deadenylation, which leads to decapping and 5′–3′ decay.
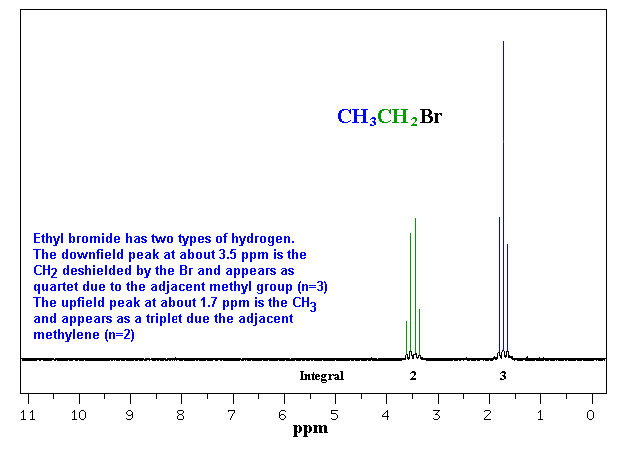
The control of mRNA degradation and translation are important for the regulation of gene expression.
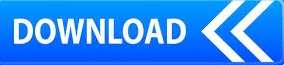